Pyroclastic Density Currents (PDCs) are ground-hugging clouds of super-heated volcanic ash, rock and gas that have a reputation for destroying everything in their path. Ranging from particle-dominated flows through to gas-dominated surges, PDC’s can be triggered by a range of volcanic events—not just large eruptions—and are responsible for more than a third of all volcano-related deaths worldwide. This makes them a top priority for research so we can develop better hazard models and provide better advice to keep people safe around active volcanoes.
“In New Zealand, all of our volcanoes produce PDCs, so one of the foremost tasks of volcanologists is to forecast and mitigate against PDC hazards,” Volcanoes Programme researcher Prof Gert Lube of Massey University told attendees at the International Association of Volcanology and Chemistry of the Earth’s Interior (IAVCEI) Scientific Assembly held recently in Rotorua.
Gert runs the Pyroclastic flow Eruption Large-scale Experiment (PELE) facility at Massey University. While the inherently destructive nature of PDCs makes them difficult and dangerous to study in the field, the PELE apparatus—the largest of its type in the world—enables Gert and his team to study PDCs in a safe and controlled environment.
“We use between 250 and 5,000 kg of volcanic ash and pumice collected from the Taupō ignimbrite, which is sieved to less than 16 mm grainsize and heated to between 120 and 500 °C to simulate a scaled-down equivalent of a real PDC,” Gert explains.
While much smaller than their real counterparts, the experimental PDCs being generated in the old boiler house on Massey University’s Palmerston North campus are strikingly realistic. Dropped from a height of seven meters into a gently inclined, 0.5 m wide channel, the hot pile of pumice and ash quickly generates a miniature pyroclastic flow that careens down the 17 m long channel, before barrelling out a large roller door to safely peter out in the cordoned off 13 m long carpark beyond. Each experimental flow is closely monitored and continuously recorded by dozens of sensors, including suites of pressure gauges and temperature probes, microphones, and cameras. It’s a finely tuned experiment that takes months to set up and seconds to run, but which yields a wealth of data.
“Field estimates strongly underestimate the destruction-causing pressure inside these flows,” says Gert. “Our experiments show that there are turbulent pressure fluctuations that far exceed the average pressure inside the flows. These turbulent fluctuations are the root cause of the partial to complete destruction to the built environment around volcanoes.”
Data from different experimental runs enables the team to determine not only pressure fluctuations inside pyroclastic flows, but also the evolving internal structure of the flows as they propagate, how their particles behave and interact with hot gases, what makes flows capable of picking up more particles from the ground as they go, how and why pyroclastic flows can propagate much further than expected, and how they interact with the confining nature of the channel walls, ambient air, and even with water.
“It takes weeks to months to set up…In our first test run we had to overcome multiple water leaks,” says PhD student Jeff Robert, who hopes to use a modified version of the PELE apparatus to investigate how pyroclastic flows can generate tsunamis. By partially filling the channel with water and installing a pool at the end, he can watch and record how a pyroclastic flow separates out into a cloud flowing across the water surface, and a submarine sediment-laden current along the seafloor.
“Our first run was to test the set up. Next time will be the real experiment,” Jeff says.
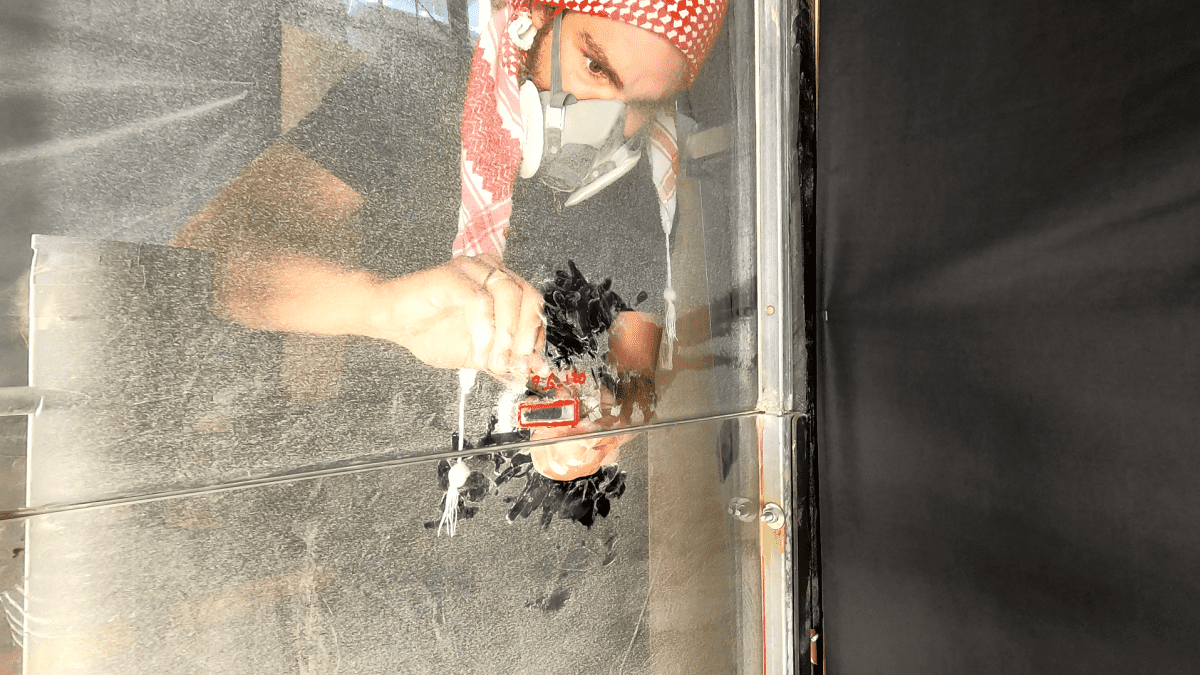
Another PhD student, Lucas Corna has investigated how pyroclastic flows respond to barriers in their path, work that has significant implications for people who might assume they are safe from a pyroclastic flow travelling down a valley if they themselves are high up on a nearby ridge.
“We found that the ratio was 5:1. You need a barrier to be five times higher than the height of the flow otherwise the flow can overtop it,” Lucas says.
Meanwhile, Anna Perttu’s PhD is seeking to determine if infrasound can be used to measure and monitor real pyroclastic flows from a safe distance.
“Infrasound is sound that is below the range of human hearing,” Anna explains. “We’re working in the lab here to determine if we can use infrasound to peer inside pyroclastic density currents to see if we can determine their physical properties in the field.”
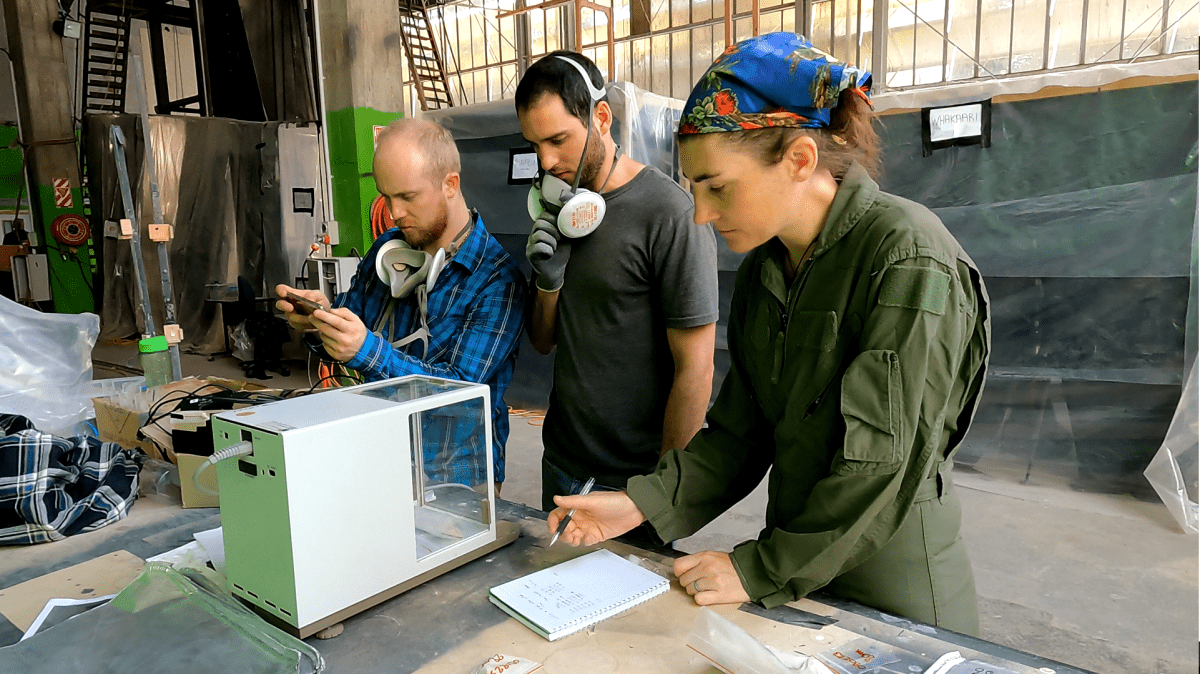
Gert and his team are also providing data to help test and validate PDC models developed by international colleagues. In the days after the IAVCEI conference, many of these colleagues embraced a 5.30am start to watch the final set up and see the PELE apparatus in action.
Through such collaborations Gert and his team are contributing to a global effort to better understand and predict the behaviour of pyroclastic flows.
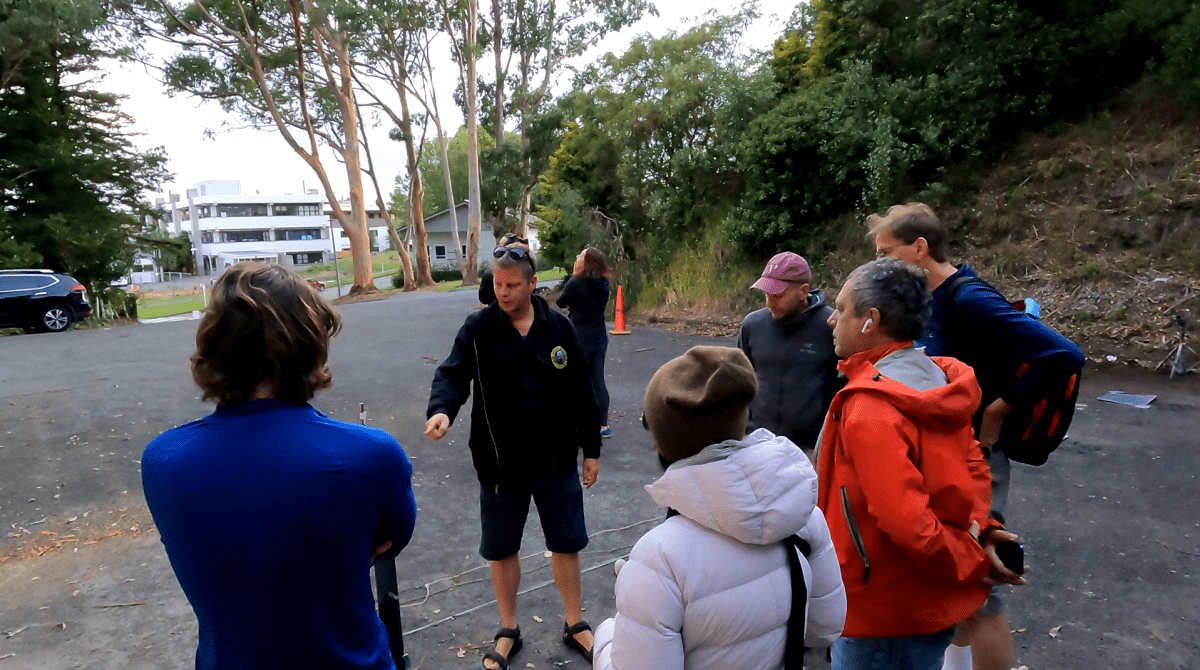