By Tim Davies, Case Study Project Leader, Multihazard Risk Programme.
The Multihazard Risk Modelling (MRM) theme of the Resilience Challenge addresses the issues of modelling coincident and cascading hazards and their consequences, including their long-term and society-wide social and economic impacts. We use realistic scenario frameworks together with improved resilience investment business cases. The main target hazard selected is that of a river sedimentation and flooding scenario in the Rangitaiki-Tarawera river system of the North Island, driven by a volcanic eruption in the Taupo Volcanic Zone which also causes seismicity, lahars and mass movements (such as landslides). Rainfall and tectonic earthquakes are added as coincident hazards.
The Case Study simulation is developing a 2-3 decade-long forecastable multihazard scenario environment to provide a testbed for hazards forecasting, impact/operability analysis, economic analysis and decision-making tools. The hazard scenario is a combination of prolonged volcanic activity, tephra deposition and erosion, and volcanic mass movements, leading to river aggradation and increased flooding. It also involves a syneruptive seismic sequence of lahars and rainfall- and earthquake-generated landsliding. The Case Study team includes Tim Davies, Mark Bebbington, Stuart Mead, Melody Whitehead and David Harte, with specialised input from Murray Hicks.
This is the first time such forward modelling of eruption-driven river behaviour has been attempted globally, and the fluvial consequences of a large eruption have not previously been studied in New Zealand. The eruptions of Mt St Helens (USA, 1981), Pinatubo (Philippines, 1991) and Chaitén (Chile, 2008) provide solid evidence that these consequences can be both severe and prolonged (Fig. 1), so it is to be expected that the societal impacts will be similarly serious.
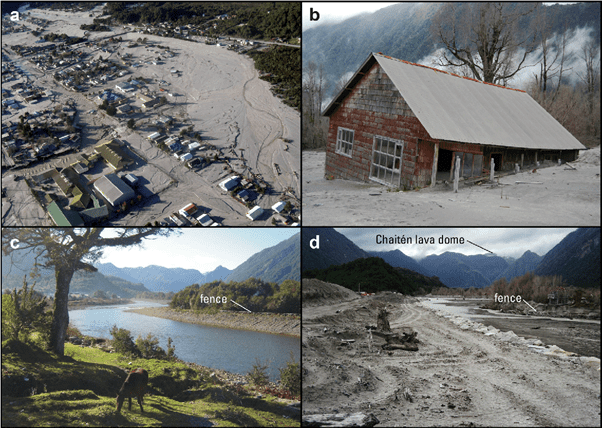
The hazard sequence of the Case Study will be initiated by a volcanic eruption and ash deposition event from the Okataina volcanic complex. This last erupted from Mt Tarawera in 1886 AD, but the simulated event will be loosely modelled on the Kaharoa event of c. 1315 AD. The simulated event will be a decade-scale eruption sequence combined with a realistic weather sequence that includes ex-tropical cyclones, all of which will be generated probabilistically. Outburst events from river blockage are also possible and will be included in the models.
The Case Study models the impacts of these events on sedimentation and flooding in the Rangitaiki-Tarawera river system, and their consequential impacts on infrastructure, society and the economy, both locally and nationally. The objective of the physical hazard model (the “Real” or REAL model, Fig. 2) is to provide information that a forecasting model can use to generate a statistically-based dataset for the operation of other models (engineering outage, socio-economic, Māori risk and decision-making).
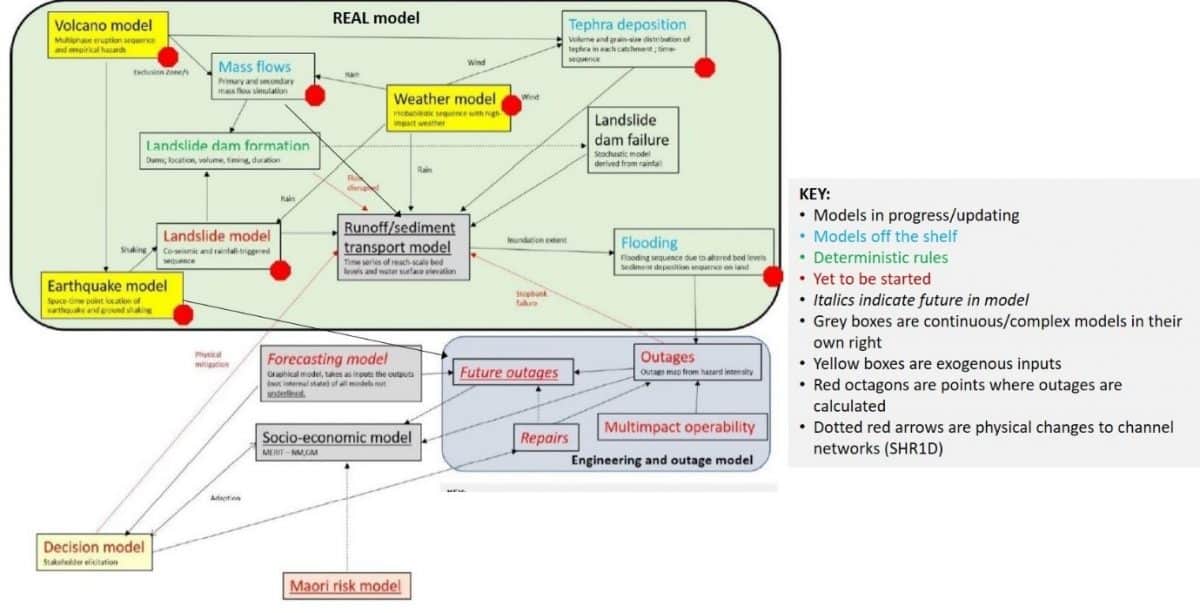
The central engine, and most complex component, of the REAL model is the Runoff/Sediment Transport Model (Fig. 3). Based on a digital elevation model overlain with geology, drainage, soils and vegetation, the REAL model uses rainfall data from 23 locations collected at 30-minute intervals, and ashfall from volcanic activity and weather models. The Non-Linear Runoff Model (NLRM) developed by Bay of Plenty Regional Council is then used to estimate rainfall runoff and river flows. Meanwhile, a calibrated version of the Modified Universal Soil Loss Equation model (MUSLE; e.g. Chalov et al., 2017) estimates tephra erosion and its transport into the river system. Finally, the one-dimensional morphodynamic model SHR1D (Greimann and Huang, 2018) simulates transport and deposition of sediment through the system with corresponding sediment deposition (aggradation) and flooding. On downstream flood-plains where aggradation can cause rivers to overtop their banks we intended to use the two-dimensional CAESAR model (Coulthard and Wiel, 2006) to represent the abandonment and formation of new river channels as flooded rivers change course.
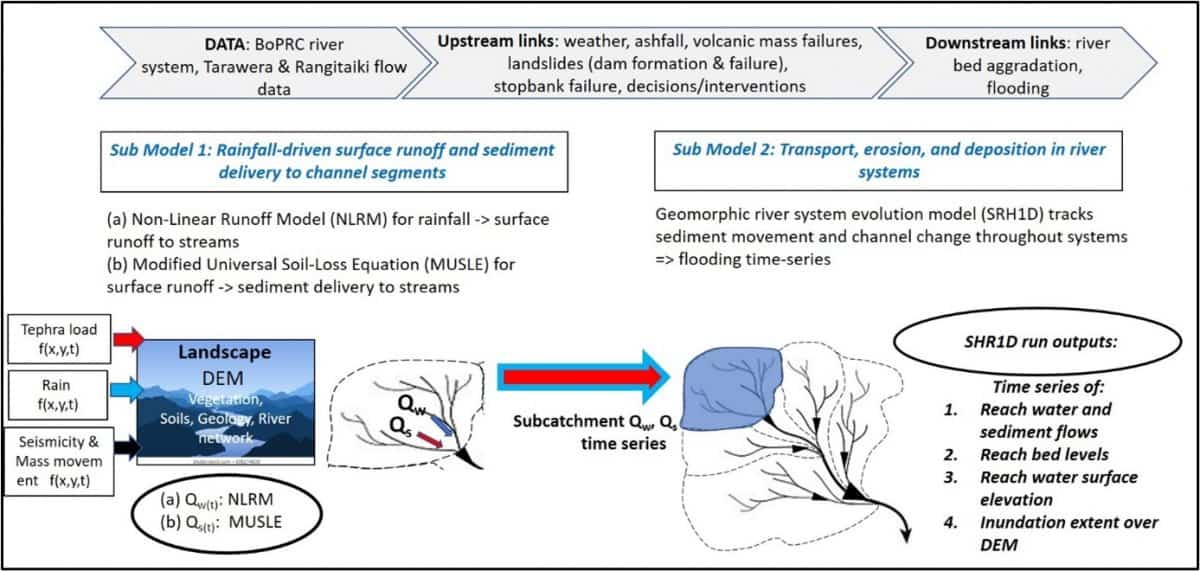
The ultimate outcome of the REAL model is a set of detailed snapshots of the sedimentation and flooding resulting from an eruption over time. The impacts of these phenomena on society can then be explored using the forecasting, engineering outage, societal, Māori risk and decision-making models.
References
Chalov, S.R., Tsyplenkov, A.S., Pietron, J., Chalova, A.S., Shkolnyi, D.I., Jarsjö, J. and Maerker, M., 2017. Sediment transport in headwaters of a volcanic catchment—Kamchatka Peninsula case study. Frontiers of Earth Science, 11(3), pp.565-578.
Coulthard, T.J. and Wiel, M.J.V.D., 2006. A cellular model of river meandering. Earth Surface Processes and Landforms, 31(1), pp.123-132.
Greimann, B. and Huang, J.V., 2018. SRH-1D 4.0 User’s Manual (Sedimentation and River Hydraulics–One Dimension, Version 4.0). Technical Report SRH-2018-07, Technical Service Center, US Bureau of Reclamation, Denver (CO), USA.
Major, J.J. and Lara, L.E., 2013. Overview of Chaitén Volcano, Chile, and its 2008-2009 eruption. Andean Geology, 40(2), pp.196-215.